2.2.1 Diet
Planning and consuming an appropriate / strategic diet are complex topics for most people, even without a chronic disease.
For those with a chronic disease, it's significantly more complex. One common challenge is selectivity - trying to ensure that your cells
are receiving these dietary nutrients, while avoiding feeding them to certain
microorganisms residing in your GI tract - which then produce endotoxins
/ mycotoxins that poison your energy metabolism and neurotransmitter
pathways. Acetaldehyde is one of the toxins discussed heavily in the disease model, as part of Gut Fermentation Syndrome (GFS).
Failure to adapt your diet around your personalised microbiome dysbiosis and chronic disease metabolism will invariably lead to "getting stuck" or even back-sliding. Unlike minerals deficiencies, which are normally slow to develop and slow to resolve, microbiome dysbiosis can rapidly shift during immune activity or by feeding the wrong microorganisms over a number of weeks. What you eat determines which microorganisms get promoted or inhibited.
Diet plays a pivotal role in chronic disease by influencing substrate availability for fermenting microbes and shaping the gut microbiota composition. High-carbohydrate diets provide ample fuel for microbial fermentation, exacerbating acetaldehyde production and metabolic disruptions. Conversely, low-carbohydrate diets can reduce substrate availability, potentially decreasing microbial fermentation and acetaldehyde levels, however these can also exacerbate glycogen storage issues and contribute to various symptoms like POTS, fatigue, depression and other neurological symptoms. This has been labelled by some as “low carb limbo”.
Depending on the functionality of different energy pathways, while first starting this protocol, you may also find you respond better to certain type of macronutrients, ie. carbohydrates, fats and proteins than others.
The performance of your diet can be predicted in the Organic Acids Test (OAT) results, by looking at the relevant pathways for metabolites of each macronutrient, eg. glycolysis, fatty acids, amino acids. Some of the adjacent pathway markers, such as ketogenesis, lactic acid and oxalate metabolism are also helpful in identifying impaired pathways, indirectly.
Therefore, before we go into detailing specific foods and their attributes, it helps to understand a little more about the different energy pathways and how dietary macronutrients can be turned into energy.
Feeding energy pathways
You need different energy substrates for different purposes and loads. Those pathways need fuels, cofactors and signalling to work correctly. Let's review them:
Instant / burst energy (muscle tissue)
ATP-PC (Phosphocreatine) System
Where: Not used in liver and adipose tissue.
Substrates: Phosphocreatine (PC) stored in muscles.
Requirements: Dietary creatine. Creatine produced from the urea cycle, using dietary protein. Dietary phosphorus intake. Promoted by sex and thyroid hormones.
Uses: High-intensity, short-duration activities (e.g., sprinting, weightlifting).
Availability / Duration: 10-15 second bursts.
Trigger/Signaling: Immediate energy demand; catecholamines increase utilization.
High intensity energy demands
Glycolysis
Where: Not used in red blood cells (RBCs), as they rely entirely on anaerobic glycolysis, and white adipose tissue prefers fatty acid metabolism.
Substrates: Glucose or glycogen.
Requirements: Dietary carbohydrate intake or gluconeogenesis. Phosphorus. Biotin. Vitamin B3 (as NAD+). Zinc. Magnesium / Manganese. Potassium. Chromium. Vanadium. Thyroxine (calcium, iodine, selenium, heme, tyrosine). Vitamin B1 (as TPP). Lipoamide. Promoted by sex and thyroid hormones.
Uses: Brain activity. Moderate to high-intensity activities (eg., walking / running upstairs, thinking).
Availability / Duration: Muscles - up to 2-3 minute bursts, subject to glycogen reserves.
Trigger/Signaling: Increased ADP levels; catecholamines enhance glucose availability via cAMP-PKA signaling, inhibiting glycogen synthase and activating glycogen phosphorylase.
Glutaminolysis (anaplerosis)
Where: Not used in red blood cells due to lack of mitochondria and adipose tissue due to limited metabolic flexibility.
Substrates: Glutamine.
Requirements: Dietary glutamine, or glutamine created from citric acid cycle activity. Vitamin B3 (as NAD+). Zinc. Magnesium / Manganese. Promoted by sex hormones.
Uses: Rapid cellular proliferation (eg., immune response) and intense brain activity.
Duration: Continuous during demand.
Trigger/Signaling: Stress-induced catecholamine elevation may indirectly upregulate this pathway.
Sustained energy demands
Fatty Acid Oxidation
Where: Not used in brain cells due to fatty acids not crossing the blood-brain barrier, nor red blood cells due to lack of mitochondria.
Substrates: Fatty acids
Requirements: Dietary fats or lipolysis. Carnitine for the carnitine shuttle. Vitamin B2 (as FAD). Vitamin B5 + cysteine (as Coenzyme-A). Lymphatic system is used to transport dietary fats.
Uses: Basal daily metabolism. Low to moderate-intensity activities (eg., walking, endurance sports).
Duration: Indefinite.
Trigger/Signaling: Prolonged energy demand; catecholamines promote lipolysis, increasing free fatty acids for oxidation.
Branched Chain Amino Acid (BCAA) Catabolism
Where: Low activity in the smooth muscle, brain and liver. Primarily used in skeletal muscle, heart, and kidney tissues.
Substrates: Leucine, isoleucine, valine.
Requirements: Dietary protein intake. Vitamin B2 (as FAD). Biotin. Vitamin B12 (as adenosylcobalamin). P5P.
Uses: Prolonged exertion, especially with low glycogen.
Duration: Sustained.
Trigger/Signaling: Catecholamines promote protein breakdown, increasing BCAA availability.
Backup energy pathways for excessive load and various mitochondrial dysfunction
Anaerobic Glycolysis / Lactic Acid Metabolism
Where: Not used in liver (under normal conditions) and heart (prefers aerobic metabolism). Promotes neurogenesis and myogenesis.
Substrates: Glucose.
Requirements: Dietary carbohydrate intake or gluconeogenesis. TBC.
Uses: High-intensity activities with limited oxygen.
Duration: Short bursts, up to 2-3 minutes.
Trigger/Signaling: High ADP/ATP ratio, catecholamines enhance lactate production through glycogenolysis.
Astrocyte-Neuron Lactate Shuttle (ANLS)
Where: Found in the brain. Lactate can also circulate and participate in the Cori Cycle.
Substrates: Glucose (astrocytes), lactate (neurons).
Requirements: Dietary carbohydrate intake or gluconeogenesis.TBC.
Uses: Neural activity, intense cognitive tasks where glycolysis is insufficient.
Duration: Continuous during brain function.
Trigger/Signaling: IFN-gamma, others. Norepinephrine enhances glucose uptake in astrocytes, promoting lactate production for neurons, similar to an intra-brain Cori cycle.
Cori Cycle / Lactic Acid Cycle
Where: Systemic
Substrates: Lactate (from muscles, brain).
Requirements: Dietary carbohydrate intake or gluconeogenesis. TBC.
Uses: High-intensity exertion / mitochondrial dysfunction.
Duration: Normally short-term, during recovery.
Trigger/Signaling: Epinephrine increases lactate production, fueling the Cori cycle.
Ketogenesis + Ketosis
Where: Ketones are generated in the liver, but not used by it. Red blood cells cannot use ketones due to lack of mitochondria.
Substrates: Fatty acids.
Requirements: Dietary fats and/or lipolysis. TBC.
Uses: Prolonged fasting, low carbohydrate intake / impaired glycolysis, or low liver glycogen.
Duration: Sustained during glycogen depletion.
Trigger/Signaling: Catecholamines promote lipolysis, increasing fatty acids for ketone body production.
Examples of some less utilised pathways, which may be promoted / abused when primary pathways fail
Tyrosine Catabolism
Where: Not used in red blood cells and adipose tissue due to limited enzymatic activity for this pathway.
Substrates: Tyrosine.
Requirements: Dietary intake of tyrosine and/or microbiome synthesis. P5P. Ferritin. Calcium. Magnesium.
Uses: Various, including stress, mitochondrial dysfunction.
Duration: Continuous, where tyrosine exists.
Trigger/Signaling: Catecholamines do not directly regulate but are derived from tyrosine.
GABA Shunt
Where: Not used in liver and skeletal muscle as they are not involved in neurotransmitter metabolism.
Substrates: GABA via glutamate or polyamine metabolism.
Requirements: P5P, magnesium.
Uses: Neural activity, especially during TCA cycle impairment and oxidative stress.
Duration: Continuous.
Trigger/Signaling: Catecholamines influence neurotransmitter release but do not directly regulate GABA metabolism.
Urea Cycle (Argininosuccinate)
Where: Not used in brain and muscle tissue since the urea cycle is primarily hepatic.
Substrates: Amino acids (arginine).
Requirements: Dietary protein intake. Aspartate.
Uses: Ammonia elevation, protein metabolism / high protein intake.
Duration: Continuous.
Trigger/Signaling: Catecholamines indirectly increase ammonia load, requiring urea cycle activity.
Food Intolerances
You also may have already developed a number of food intolerances.
Some of these intolerances are "simply" endotoxins being produced by overgrowth of unwanted microbial species living in biofilms inside your GI tract, consequently being sustained by your intake of various dietary carbohydrates and/or other nutrients, such as sulfur-rich compounds These endotoxins can inhibit energy metabolism, neurotransmitter homeostasis, histamine degradation and various other pathways.
Standard mixed diets vs ketogenic diets
"Sometimes the easiest way to solve a problem is to stop participating in the problem." - Unknown
(In this context, "You cannot efficiently eliminate or reduce overgrown species while you're also feeding them.")
Standard mixed diet
A standard mixed diet typically includes a balanced intake
of carbohydrates, proteins, and fats. In the context of the disease model, this diet may
inadvertently contribute to increased fermentation and unwanted biofilm development. The high carbohydrate
content fuels microbial ethanol and acetaldehyde production, leading to
continued impairment of energy pathways due to elevated acetaldehyde. Symptoms
such as fatigue, cognitive impairment, and metabolic abnormalities may persist
or worsen under this dietary regimen.
Depending on the species and locations of the biofilms
containing these microbial species, you may have more success avoiding
symptoms by consuming different types of carbohydrates.
eg.
a.
Simple carbohydrates / sugars may feed any existing microbial
overgrowth in the mouth / throat / oesophagus / stomach / small
intestine. In the absence of dysbiosis in those tissues, simple
carbohydrates can also be readily absorbed in the small intestine,
before reaching any dysbiosis in the large intestine, should it exist.
b.
Complex carbohydrates may not be broken down until further along the GI
tract and may skip feeding some of the populations residing in biofilms
in the small intestine.
c. Different types of dietary fibres and starches may selectively feed some species and not others. (see "2.2.3 -- Prebiotics"")
d.
Gliadin in gluten may generate an immune response due to molecular
mimicry of the protein in the "hook" of Candida's hyphae form. Gliadin
is also used by a particular pathogenic strain of Streptococcus
salivarus to produce an antigen that punches holes in enterocytes,
leading to gut barrier degradation, immune response and inflammation. Giardia infections are also known to create gluten intolerance.
https://www.ncbi.nlm.nih.gov/pmc/articles/PMC4368562/
https://pubmed.ncbi.nlm.nih.gov/22617359/
In some types of more severe dysbiosis (and where your B vitamin and mineral status also supports this), you may find swapping more carbohydrates for
protein can be helpful in avoiding endotoxemia symptoms such as
nausea, bloating, diarrhoea, histamine reactions, tachycardia,
headaches, etc. These will resemble die-off symptoms, as the same toxins
are involved (see: 2.3.1-Herxheimer / die-off / acetaldehyde-support).
Here is an example 2000kcal mixed-diet, visualised in Cronometer:
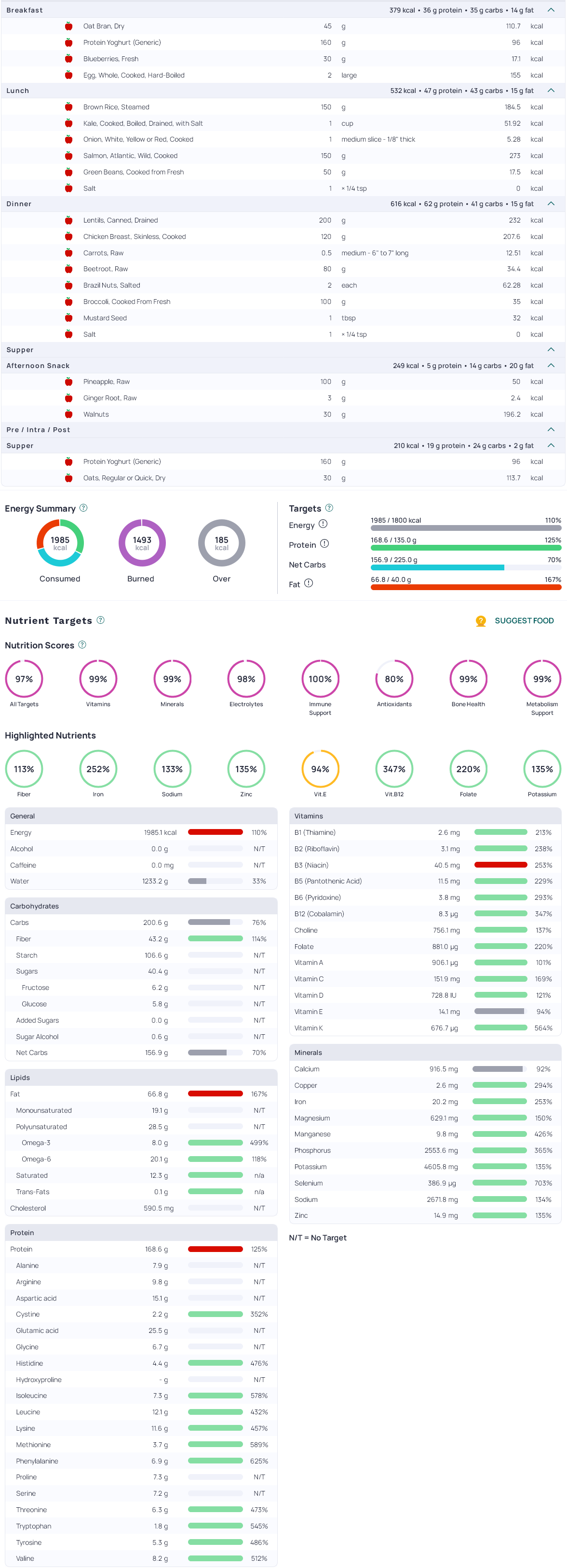
Ketogenic diet
The ketogenic diet, characterized by high fat, moderate
protein, and very low carbohydrate intake, offers a contrasting approach to
a standard mixed diet or a low carbohydrate diet.It also avoids "low carb limbo" by promoting generation of ketone bodies in the liver.
In extreme gut microbiome dysbiosis cases, changing to a
ketogenic diet for a period of time can also be very helpful in reducing
fermenting species, however will require commitment to endure an often
unpleasant induction (1 week) and then adaptation phase (2 weeks). If
your OAT results already show elevated ketone markers, this is much
easier. Studies suggest the acute effects of a ketogenic diet on your
gut microbiome will last around 12 weeks, before the microbiome adapts.
Combining this window of opportunity with other interventions in this protocol can allow
you to break the existing cycle with lasting results.
By significantly reducing carbohydrate consumption, this diet limits the
substrates available for microbial fermentation. As a result, endogenous
ethanol and acetaldehyde production may decrease significantly in response to food
intake, alleviating some of the metabolic disruptions caused by GFS.
However, circulating acetaldehyde and other endotoxins will still be
experienced, making the first 1-2 weeks of a ketogenic (the induction phase)
more unpleasant than it would be for someone without GFS.
A key point of difference is that the ketogenic diet promotes fatty acid oxidation and ketone body production as alternative energy sources. Ketone bodies can cross the blood-brain barrier and provide energy to the brain (much more efficiently after weeks of being on a ketogenic diet), potentially improving cognitive function and reducing fatigue. Additionally, this diet may alter the gut microbiota significantly, reducing fermenting microbial populations and promoting a healthier balance.
Mechanisms of Ketogenic Diet's Impact on GFS
Carbohydrate Restriction
Limiting carbohydrate intake directly reduces the amount of glucose and other fermentable sugars in the gut. This reduction in substrates can lead to decreased microbial fermentation activity, resulting in lower ethanol and acetaldehyde production. With less acetaldehyde burden, the inhibitory effects on metabolic enzymes are alleviated, helping to restore the cellular redox balance and improve the function of gluconeogenesis and fatty acid oxidation pathways.
Fatty Acid Oxidation and Ketogenesis
The ketogenic diet's high-fat composition encourages the body to utilize fats as the primary energy source. This shift promotes the production of ketone bodies in the liver through a process called ketogenesis. Ketone bodies serve as an efficient alternative energy source for various tissues, including the brain and muscles. By providing a consistent energy supply, the diet addresses the energy deficits caused by acetaldehyde-induced metabolic disruptions. Additionally, relying less on impaired glucose production pathways reduces the risk of hypoglycaemia.
Benefits of a Ketogenic Diet in GFS
Implementing a ketogenic diet in GFS management offers
several potential benefits. By reducing acetaldehyde levels, the diet can help
restore normal metabolic functions, improving energy production and overall
well-being. The provision of ketone bodies as an alternative energy source may
alleviate symptoms such as fatigue and cognitive impairments. Furthermore, the
reduction in carbohydrate intake may lead to favourable shifts in gut
microbiota composition, decreasing the population of fermenting microbes
responsible for excessive acetaldehyde production.
Potential Challenges
Despite its potential advantages, the ketogenic diet
presents challenges that must be carefully considered. The restrictive nature
of the diet can make long-term adherence difficult for some people. There is
also a risk of nutrient deficiencies, particularly in electrolytes, fibre,
vitamins, and minerals, if the diet is not properly managed. Monitoring thyroid
function is also helpful, as very low carbohydrate intake can sometimes reduce
T3 levels, potentially affecting metabolic rate. Additionally, high-fat diets
can impact beneficial gut bacteria, necessitating careful planning to maintain
gut health.
A manganese deficiency will significantly impact hepatic gluconeogenesis, as will zinc and magnesium deficiencies.
These should be somewhat addressed before attempting keto induction.
NB. The reduction in glucose availability may affect cognitive function where neural hypoxia exists - due to eg. structural issues, coagulation and/or transport issues, severe iron deficiency, and also blocked lymph nodes in the neck and/or nasopharynx (usually relating to localised infection). The reduced glucose affects lactic acid metabolism involved in the Astrocyte-Neuron Lactate Shuttle and ultimately energy availability in neurons.
Strategic Implementation
Successful implementation of a ketogenic diet for GFS
requires proper planning to ensure safety and efficacy. A personalised
nutritional plan tailored to individual needs and tolerances can enhance
adherence and effectiveness. Cronometer
is very helpful in planning and visualising a successful day. Setting expectations ahead of time will help allow you to "keep calm and keto on" during unpleasantness.
Supplementation with essential electrolytes, vitamins, minerals, and probiotics
like the ones in this protocol may be necessary to address deficiencies and
support gut health.
A gradual transition into the diet, by slowly reducing carbohydrate intake, prolongs
keto adaptation and exacerbates energy crisis symptoms. It is not recommended.
A rapid transition normally creates temporary symptoms of "keto flu",
which can be managed by using GoBHB exogenous ketone supplements and essential
amino acids (EAAs) during the dietary transition.
Strictly restricting carbohydrate intake is paramount to the
diet, which involves eliminating sugars and refined carbohydrates like
sweets, sugary drinks, white bread, and pastries. Removing starchy foods
such as potatoes, rice, pasta, and grains is also necessary. Instead, consuming
non-starchy vegetables like broccoli, cauliflower, kale, and bell peppers (capsicum)
provides essential nutrients with minimal carbohydrates. The target daily net
carbohydrate (total carbohydrates, minus fibre) upper limit is 25g.
Increasing the intake of healthy fats supports the
ketogenic approach. This includes consuming monounsaturated fats found in olive
oil, avocados, and nuts, as well as omega-3 fatty acids from fatty fish such as
salmon and mackerel. Saturated fats from sources like coconut oil and butter
can be included. Butter is also a good source of butyrate, which may trigger rapid,
beneficial changes to the microbiome, however, this will also trigger die-off
symptoms and potentially induce rapid alcohol withdrawal symptoms.
Protein intake is important for essential amino acids and to
allow the liver to perform hepatic gluconeogenesis for brain and other tissues.
Balancing protein intake is important because excessive protein can be
converted into glucose, potentially triggering an insulin response that
inhibits keto induction / adaptation, while also fuelling microbial fermentation,
depending on the location of the biofilms. The longer the protein take to
digest, the further along the GI tract it will be before potentially feeding
the microbiome.
Keto induction is easier with more fats than protein, initially.
For example,
During keto induction, you could consume:
170g fat @ 1530 kcal +
100g protein @ 400 kcal
= 1930kcal
After keto induction, you can transition to consuming roughly 1:1 of fat:protein, on a per-gram
basis:
150g fat x9 = 1350kcal
150g protein x4 = 600kcal.
= 1950kcal.
(Scale this by your calorific needs.)
High-quality protein sources such as lean meats, poultry, eggs (a great source of
lecithin for phosphatidylcholine), and plant-based proteins like nuts (good
mineral sources) and tofu (some people may have tolerance issues) are
recommended.
Maintaining proper hydration and electrolyte balance is
essential. Adequate water intake supports detoxification and metabolic
processes, while sufficient electrolytes help prevent imbalances that will
reliably occur during the initial stages of a ketogenic diet, as glycogen
stores are intentionally depleted.
Here is an example 2000kcal ketogenic diet, visualised in Cronometer:
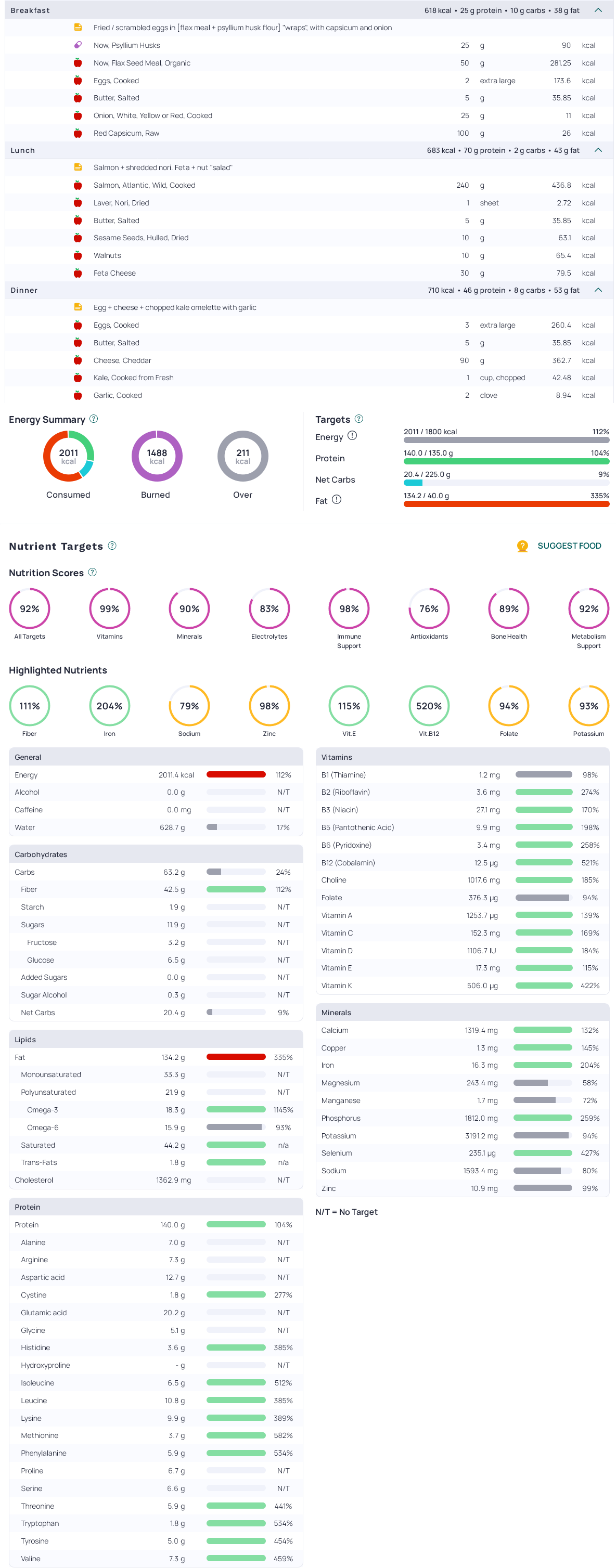
Inflammation and/or gut membrane permeability issues due to microbiome dysbiosis and related immune activity often leads to further histamine elevation and temporary IgG antibodies being produced. This is normal behaviour in response to complete foreign proteins getting through the barrier and triggering an immune response. In this state, foods which trigger mast cell activation and histamine release should be avoided for 6-8 weeks before reintroducing them, preferably after the barrier dysfunction has been sufficiently improved / resolved.
Due to metabolic impairments being described in this disease model, other food intolerance issues are entirely expected. The disease model and collected data shows that aldehyde toxicity / impaired aldehyde metabolism leads to elevations of serum Vitamin B6, creates issues metabolising "inactive" forms of Vitamin A into the active forms, creates issues degrading histamine and separately, other issues promoting lactic acid metabolism also elevate endogenous biosynthesis of oxalate.
In this state, additional intakes of these nutrients / compounds may exacerbate existing symptoms. These issues are targeted in Stage 1 of the protocol and allows dietary intakes to become more flexible. Overall, foods need to be carefully managed and reintroduced as the related
metabolic impairments and barrier function issues are resolved.
Active Vitamin A deficiency
Vitamin A isn't a single molecule, but rather a chain of metabolic conversions that transform dietary precursors into active signalling compounds. The story begins with retinyl esters, the storage form found in the liver and other tissues. When needed, these are converted into retinol, a transportable alcohol that circulates through the bloodstream and enters cells as required. (see Figure 8)
Inside the cell, retinol takes on a more active role. It's oxidised into retinaldehyde (often shortened to retinal) and then further into retinoic acid, the bioactive form that influences gene expression. These transformations are not spontaneous. They are mediated by specific enzymes, and each one depends on particular cofactors and cellular conditions.
There are several isomers involved in this process. The most common is all-trans-retinol, which feeds into the production of all-trans-retinoic acid (atRA). But there are also 9-cis and 11-cis forms of both retinol and retinal, each with their own signalling roles. The final step - the irreversible conversion of retinal into retinoic acid - is catalysed by the aldehyde dehydrogenase enzymes: primarily ALDH1A1, with ALDH1A2 and ALDH1A3 playing secondary roles depending on the tissue. This is the same enzyme responsible for degrading neurotransmitters.
This is where problems begin to surface. ALDH1A1 is a fragile enzyme. It requires NAD⁺ to function, which makes it vulnerable in states of redox imbalance. Conditions that lower NAD⁺, such as mitochondrial dysfunction, acetaldehyde excess, inflammation, or chronic infection-can slow the enzyme down. Worse, acetaldehyde, a byproduct of both alcohol metabolism and microbial fermentation, also directly inhibits ALDH1A1. Add in common deficiencies of zinc and magnesium, or a cellular environment overloaded with oxidative stress, and the enzyme’s activity may be effectively crippled.
When ALDH1A1 fails, the metabolic pathway clogs. Retinal cannot be converted into retinoic acid. As this step is irreversible, there’s no workaround within the body. Retinol supplements won't help - in fact, retinol can build up to apparently toxic levels. Carotenoids won't help. The machinery is blocked downstream, and the most critical signals simply don’t arrive.
Retinoic acid itself exists in several forms. All-trans-retinoic acid activates retinoic acid receptors (RARs) that regulate genes responsible for epithelial integrity, preventing hyperkeratosis (KLK pathway), immune regulation, detoxification, and neurodevelopment. Meanwhile, 9-cis-retinoic acid is required to activate retinoid X receptors (RXRs). RXRs are the central dimerisation partners for many other nuclear receptors, including PPARs, LXR, FXR, VDR, TR, and others. Without 9-cis-retinoic acid, these systems cannot operate at full capacity.
The consequences of low 9-cis-RA reach far beyond vitamin A metabolism. RXR-PPAR dimers, which govern fatty acid oxidation, ketogenesis, lipoprotein metabolism, adipogenesis, insulin sensitivity, inflammation resolution, energy expenditure, muscle metabolism and neuroprotection become hypoactive.
RXR-LXR dimers, essential for cholesterol transport and immune homeostasis, stall. Even RXR-VDR complexes, which modulate calcium and vitamin D responses, lose efficacy. FXR heterodimers regulating bile acid synthesis inhibition, plus lipid & glucose metabolism start fail to perform.
The deficiency of this one vitamin's metabolites sends a ripple through every metabolic and endocrine axis it touches.
When this problem is recognised, the logical therapeutic step, while correcting the upstream influences, would be to provide retinoic acid directly, bypassing the broken ALDH enzymatic activity. However, rather than being available as a dietary supplement, all-trans-retinoic acid is sold as a drug under the name Tretinoin. It’s used clinically for certain types of acne, photoaging, and acute promyelocytic leukaemia. But it is also a prescription-only, regulated substance, patented and restricted, despite being identical to a molecule the body produces endogenously.. when it can.
Your doctor may provide a prescription for off-label use of a tretinoin cream (not to be mistake for isotretinoin / 13-cis retinoic acid), which can be used inside a mucosal tissue targeting a daily dose of around 1mg, which might be an amount of cream resembling somewhere between a "grain of rice" and the "size of a pea", depending on the concentration of the cream. typically 0.05 - 0.1%.
There’s something quite peculiar about how a key developmental and repair vitamin which determines the behaviour of epithelial cells, immune tolerance, and even neural differentiation is, for most people, functionally inaccessible unless they're being treated for a specific, medically recognised condition. Its dietary replacement has been patented, monetised, and regulated.
In situations where ALDH1A1 is impaired-through eg. chronic alcohol exposure, microbial fermentation, or long-term metabolic damage-this silent blockade may contribute to a breakdown in tissue maintenance, mucosal immunity, and metabolic feedback. It is a central mechanism, rarely measured, yet fundamental to the organism's capacity to respond to stress, repair damage, and regulate its own biochemistry.
Meal composition and timing is also important when energy metabolism is impaired. Part of the metabolic cascade includes type-2 diabetes and metabolic syndrome, so the dietary strategies will look very similar.
In the context of the disease model including glucose uptake and glycogen storage issues, the BCAA content of dietary protein intake may be very helpful in refilling glycogen stores via an alternate route, depending on your manganese, zinc, magnesium, biotin, B1, B2, B3, P5P and B12 status. In addition to general energy availability improvements, the model shows this is helpful for preventing PEM and crashes, providing oxidative stress and coagulation is being managed.
Vitamin Q deficiency
A healthy microbiome normally produces queuine or "Vitamin Q", which has been identified as an essential nutrient (our bodies can't make it). Vitamin Q is needed for maintaining BH4, aspartic acid, asparagine, histidine, and tyrosine. It's required for mitochondrial metabolism, blood-flow and neurotransmitter homeostasis. Vitamin Q also promotes lactobacillus species. Coconut water is a surprisingly rich source. Milk and particularly wheat germ (gluten source) are also rich sources.
Strategic foods
A table of microbiome transforming foods is provided in 2.2.3 -- Prebiotics and should be considered a required part of the protocol and any strategic diet.
The concept of "roughage" and its role in helping to disrupt and remove mucous + biofilms, especially when combined with additional biofilm breaking food items / compounds is often underappreciated in meal planning.
Foods to generally consume as part of the protocol (as tolerance allows / increases):
Foods to consume cautiously (or in some cases, avoid) as your aldehyde metabolism, etc normalises (monitor serum cholesterols, B6, beta-carotene):
In the tables below, you'll find food items and their relevant attributes, which you can use to help you get started building meals.
These tables are best viewed on a computer or tablet. On mobile devices, some columns will be hidden to fit the screen.
The columns can be sorted and cautions or elevations of potentially inappropriate nutrients in foods items when getting started have been highlighted in red.
These tables are not intended to replace https://cronometer.com, which is incredibly helpful in visualising and planning your dietary strategy.
Protein sources:
Carbohydrate sources:
Micronutrient sources: